Abstract:
In this study, commercial nata de coco was used as a high-purity bacterial cellulose resource to easily prepare aerogels via freeze-drying. The physical properties of the aerogels were investigated via the employment of X-ray diffraction, scanning electron microscopy, thermogravimetric analysis, Fourier transform infrared spectroscopy, and nitrogen physisorption measurements at 77 K. It was observed that the as-prepared cellulose aerogels possess a three-dimensional network with high porosity, high purity, and ultralight density. Therefore, this biomaterial exhibits high prospects in a myriad of applications, such as environmental treatment or thermal and sound insulation.
Keywords: bacterial cellulose, aerogel, commerical nata de coco, freeze drying.
1. Introduction
Bacterial cellulose (BC) has been employed for diverse applications in biotechnology, medicine and cosmetics [1, 2]. Such interest results from a fermentation-based high-quality and cost-effective cellulosic material which possesses favorable properties such as high crystallinity, high water retention capacity, and a porous matrix [3, 4]. Notably, different from plant-derived cellulose, BC is free from lignin, hemicellulose and other biopolymers; therefore, no toxic chemicals and no complicated processes were needed to isolate and purify bacterial cellulose [1].
Moreover, the fibrous network of BC is naturally generated in a hydrogel-like form containing a great amount of water (up to 99 wt.%) [3, 5]. It was reported that the properties of BC such as morphology, porosity, and mechanical strength are tremendously affected by the drying strategies.
In particular, air drying and oven drying, which are the most conventional methods for the removal of water from materials, can lead to the collapse of the original porous structure of the bacterial cellulose network, affording a thin and rigid sheet [5, 6].
On the other hand, freeze drying in which water is precedingly frozen and subsequently sublimated enables to maintain porosity owing to the lower surface tension caused on the cellulose matrix [6]. The successful replacement of solvents such as water with air results in the formation of so-called aerogels with 95% air and 5% network structure [7].
Indeed, lightweight aerogels derived from nata de pina and nata de coco, which were prepared in laboratory via the fermentation of pineapple extract and coconut water, respectively, were successfully obtained via utilizing the freeze-drying method. The surface area of approximately 35 m2/g was reported for these aerogels, which exhibited an excellent performance in removing organic dyes from aqueous medium [6, 8].
In the present work, commercial nata de coco the bacterial cellulose aerogel was obtained via freeze-drying. The structural characteristics of as-synthesized BC-derived aerogels were analyzed in terms of crystallinity, morphology, and thermal stability. The effect of BC concentration in the aqueous suspension on the density and porosity of the obtained aerogels was also examined.
2. Experimental section
2.1. Preparation of the BC aerogel from commercial nata de coco
Figure 1. Commercialized nata de coco pieces (a), suspension mixture of ground nata de coco and water (b), and resulting BC aerogel (c)
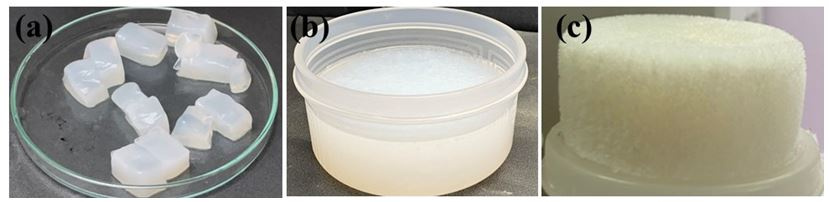
Source: Results obtained by the authors
Nata de coco was commercially obtained from the Bich Lien Duong supplier (Ben Tre, Vietnam) (Figure 1a). Nata de coco pieces (100 g) were mixed with water (100 g). A Philips HR2531 hand-blender (650 W) was employed to grind the mixture for 3 minutes and water was subsequently added to the mixture or removed from the mixture to obtain a suspension phase with a predetermined nata de coco content (10 to 90 wt.%) (Figure 1b).
The resulting suspension mixture in sealed propylene boxes were frozen at −20 °C for a day. The bacterial cellulose aerogels were obtained by freeze-drying the frozen samples, affording cylinder-shaped aerogels (Figure 1c), which was denoted based on the weight content of nata de coco in the suspension mixture, namely 10, 30, 50, 70 and 90.
2.2. Characterization of the obtained BC aerogels
Crystallinity of bacterial cellulose in nata de coco was analyzed by X-ray diffraction (XRD) on a D8 Advance diffractometer instrument (Bruker, Germany, Cu radiation). Fourier transform infrared (FT-IR) spectroscopy was performed on a Bruker Vertex 70 spectrometer (Bruker, Germany). Scanning electron microscopy (SEM) images of the aerogel were achieved on a S-4000 microscope (Hitachi, Japan). Thermal behavior of bacterial cellulose was discovered by thermogravimetric analysis (TGA) on an SDT Q600 Thermal Gravimetric Analyzer (TA Instruments, USA). Textural properties of bacterial cellulose were determined by isothermal sorption measurements with nitrogen at 77 K using an ASAP 2020 equipment (Micromeritics, USA).
3. Results and discussion
Unlike plant cellulose which contain numerous impurities such as lignin and hemicellulose, BC is fabricated in a high purity via Acetobacter xylinum-based fermentation of natural or artificial carbohydrate sources. In Vietnam, nata de coco with 0.8-1 wt.% of BC has been widely produced from coconut water. The crystalline structure of the aerogel obtained from the suspension phase containing 10 wt% of commercial nata de coco was observed in the XRD result (Figure 2a). The obtained pattern demonstrated the characteristics peaks at 2q = 14.6, 16.9, and 22.7° corresponding to (1 0), (110) and (200) lattice planes of the crystalline cellulose phase, respectively, which was in good accordance with previous studies on the cellulose obtained from the bacterial pathway [6, 8, 9].
The FT-IR analysis could be applied to indicate organic bonds present in the cellulose chain (Figure 2b). Many vibration bands, namely at 3340 cm−1 (O-H stretching), 2895 cm−1 (C−H stretching), and 1056 cm−1 (C−O bending), were observed in the spectrum of the BC aerogel which are attributed to the typical functional groups of cellulose [6].
In addition, it was confirmed that the as-produced bacterial cellulose was devoid of lignin and hemicellulose, which are typically present in the plant-derived cellulose [5].
Figure 2. XRD pattern (a), FT-IR spectrum (b), SEM image (c) and TGA profile (d) of the BC-based aerogel
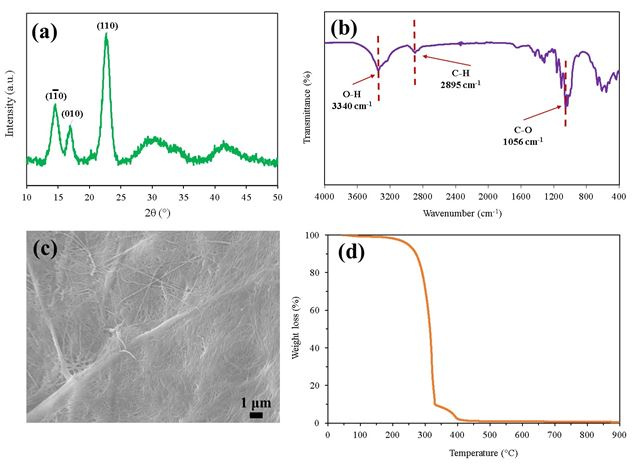
Source: Results obtained by the authors
SEM analysis was conducted to investigate the morphological characterization of cellulose in the aerogel (Figure 2c), showing an irregular three-dimensional cellulose network, which was produced by A. xylinum randomly located in the static coconut water medium [6, 8, 10]. Furthermore, it can be observed that the framework included nanofibers (< 100 nm) interconnected through inter- and intra- molecular hydrogen bonding, affording a large number of small capillaries [3]. Thermal stability of BC aerogel was evaluated via TGA (Figure 2d). The obtained TGA profile showed that the thermal degradation process of the BC aerogel involved two main weight loss stages. A slight weight loss (5%) from 50 to 150 °C, indicating the evaporation of the water [11]. The second significant weight loss occurred in the temperature range of 200 - 450 °C upon dehydration, oxidation and combustion of the cellulose matrix, releasing water, carbon oxides and other volatile products [12]. Over the temperature of 800 °C, the material was completely combusted, proving the high purity of cellulose prepared from the bacterial fermentation.
Figure 3. Density and porosity (left) and N2 sorption isotherms (right) of the BC aerogels
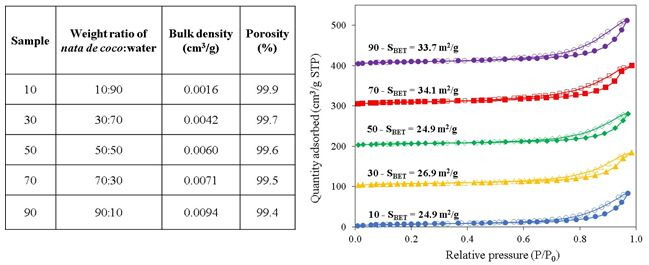
Source: Results obtained by the authors
Density and porosity of the obtained bacterial cellulose based - aerogels play a key role in determining the efficiency of the aerogel preparation by freeze-drying in order to eliminate water from the nata de coco hydrogel form (Figure 3) [5]. The freeze-drying process minimized the surface tension as no liquid droplets were involved. Therefore, the cellulose matrix can be maintained with numerous capillaries [3, 13].
As can be expected, the as-prepared aerogels possessed very low bulk density ranging from 0.0016 to 0.0094 cm3/g, depending on the nata de coco amount applied [5, 10]. This indicated the successful manufacture of such lightweight aerogels. In the study scope, the calculated high porosity of the BC aerogels was almost unchanged (≥ 99.4%) although the structure was denser by varying the nata de coco concentration from 10 to 90 wt.%.
Upon the nitrogen adsorption/desorption isotherms obtained at 77 K for the aerogel with the varied content of nata de coco (Figure 3), it could be observed that increasing in the concentration of BC in the aqueous suspension from 50 to 70 wt.% led to the enhanced specific surface areas of the as-prepared aerogels. Applying nata de coco contents lower than 70 wt.% generally yielded surface areas of about 25 m2/g while the aerogel exhibited higher surface areas of about 34 m2/g at the nata de coco concentrations of 70 and 90 wt.%. This trend can be explained based on the presence of more BC fibers in the same volume, which can form more pores, increasing the surface area.
4. Conclusions
In summary, the hydrogel-like nata de coco constructed with numerous high-quality nano-scale cellulose fibers via bacterial pathway was further utilized freeze drying method to form 3-D porous cellulose aerogel framework. The unique structure of the bacterial cellulose-based aerogel provides interesting properties, namely high porosity, high purity, and biodegradability. Valorization of carbohydrate resources and the rationalization of aerogel preparation exhibit the expectation for broader application of this such abundantly available biomaterial.
Acknowledgements:
This research is funded by Ho Chi Minh City University of Technology (HCMUT), VNU-HCM under grant number SVKSTN-2023-KTHH-09.
References:
1. Iguchi, M., S. Yamanaka, and A. Budhiono (2000). Bacterial cellulose - a masterpiece of nature's arts. Journal of Materials Science, 35(2), 261-270.
2. Lopes, T.D., et al. (2014). Bacterial cellulose and hyaluronic acid hybrid membranes: Production and characterization. International Journal of Biological Macromolecules, 67, 401-408.
3. Ul-Islam, M., et al. (2013). Effect of post-synthetic processing conditions on structural variations and applications of bacterial cellulose. Cellulose, 20(1), 253-263.
4. Gea, S., et al. (2011). Investigation into the structural, morphological, mechanical and thermal behaviour of bacterial cellulose after a two-step purification process. Bioresource Technology, 102(19), 9105-9110.
5. Illa, M.P., C.S. Sharma, and M. Khandelwal (2019). Tuning the physiochemical properties of bacterial cellulose: effect of drying conditions. Journal of Materials Science, 54(18), 12024-12035.
6. Le, H.V., et al. (2023). Bacterial Cellulose Aerogels Derived from Pineapple Peel Waste for the Adsorption of Dyes. ACS Omega, 8(37), 33412-33425.
7. Zhou, T., et al. (2019). Mechanical performance and thermal stability of polyvinyl alcohol–cellulose aerogels by freeze drying. Cellulose, 26(3), 1747-1755.
8. Phan, H.T., et al. (2023). Nata de coco as an abundant bacterial cellulose resource to prepare aerogels for the removal of organic dyes in water. Bioresource Technology Reports, 24, 101613.
9. Khan, H., A. Kadam, and D. Dutt (2020). Studies on bacterial cellulose produced by a novel strain of Lactobacillus genus. Carbohydrate Polymers, 229, 115513.
10. Mohamed, M.A., et al. (2017). An overview on cellulose-based material in tailoring bio-hybrid nanostructured photocatalysts for water treatment and renewable energy applications. International Journal of Biological Macromolecules, 103, 1232-1256.
11. Mohammadkazemi, F., M. Azin, and A. Ashori (2015). Production of bacterial cellulose using different carbon sources and culture media. Carbohydrate Polymers, 117, 518-523.
12. Yang, H., et al. (2007). Characteristics of hemicellulose, cellulose and lignin pyrolysis. Fuel, 86(12), 1781-1788.
13. Tang, W., et al. (2010). The influence of fermentation conditions and post-treatment methods on porosity of bacterial cellulose membrane. World Journal of Microbiology and Biotechnology, 26(1), 125-131.
Chuẩn bị và phân tích aerogel cellulose vi khuẩn từ thạch dừa thương mại
TS. Lê Vũ Hà1,2*
Hồ Bảo Nghi1,2
Nguyễn Anh Quân1,2
Nguyễn Bá Phúc1,2
Trần Trương Bảo Ngọc1,2
TS. Nguyễn Đăng Khoa1,2
Phan Tuấn Hoàn1,2
1Khoa Kỹ thuật Hóa học, Trường Đại học Bách Khoa Thành phố Hồ Chí Minh
2Đại học Quốc gia Thành phố Hồ Chí Minh
Tóm tắt:
Trong nghiên cứu này, thạch dừa thương mại đã được sử dụng như một nguồn cellulose vi khuẩn chất lượng cao để tạo thành vật liệu aerogel siêu nhẹ dễ dàng bằng phương pháp sấy đông khô. Các tính chất vật lý của aerogel thu được đã được nghiên cứu bằng các kỹ thuật khác nhau bao gồm nhiễu xạ tia X, kính hiển vi điện tử quét, phân tích nhiệt trọng lượng, quang phổ hồng ngoại biến đổi Fourier và hấp phụ đẳng nhiệt nitrogen ở 77 K. Aerogel từ thạch dừa thương mại sở hữu mạng lưới ba chiều không có trật tự với độ xốp và độ tinh khiết cao, và tỷ trọng siêu nhẹ. Do đó, vật liệu sinh học này có nhiều triển vọng trong các ứng dụng xử lý môi trường, cách âm, cách nhiệt.
Từ khóa: cellulose vi khuẩn, aerogel, thạch dừa thương mại, sấy đông khô.